I call it “George’s Wood” because George invented it. It’s a fibrous composite with a cunning structure – just like wood. Wood is made of long tubular cells arranged in parallel; in the walls of the cells nanofibrils of cellulose, embedded in lignin, wind helically around a central void.
In the early 1970s George Jeronimidis, working with Jim Gordon at Reading University, made models of wood with tubes of helically wound glass fibres, impregnated with resin.
The fibre winding was at 15° to the long axis of the tube; not only is this angle commonly found in wood cells, but George’s analysis showed that it’s the sweet spot in the trade-off between longitudinal stiffness and strength and the work of fracture. In tension the fibres in the tube wall tend to rotate in line with the strain until the resin between the fibres fails in shear. This allows the fibres to reorientate and the tube to extend with the fibres still supporting a load. This pseudoductility is remarkable since glass and epoxy (and cellulose and lignin in wood) are brittle.
The tubes buckle inwards and pull away from each other. In wood this increases the area of the fracture surface to at least 200 times the nominal section area. These mechanisms result in graceful and tough failure. A plate of George’s Wood in a drop-weight test had specific energy absorption five times greater than toughened steel. Like wood, you can join it with nails or screws and inject resin down the tubes to repair damage. A solid sheet of carbon-fibrereinforced polymer shattered when a bullet was fired into it. George’s Wood held the bullet and had 85-90% residual strength. As body armour you can stab it with a knife – but you can’t pull the knife out for further thrusts. The tubes in George’s Wood can be made with any fibre or mixture of fibres and at different sizes, depending on the threat. The overall properties arise from structure, not from specific materials; the important factor is the angle between the fibres and the longitudinal voids – when the tube wall buckles or bends it needs somewhere to go; the voids are integral to the behaviour.
It is apparent that this material, based on the morphology found in biology, relies heavily on structure. Is this reflected in the use of resource? The graphs below show such differences between technology (the upper graph) and biology (the lower one).. They were each generated from several hundred data points derived from the internet and from research papers. They show the proportion of problems resolved by each of six parameters (vertical axis) at sizes ranging from molecular to topographic (horizontal axis). The data are imprecise. Even so the two graphs are very different.
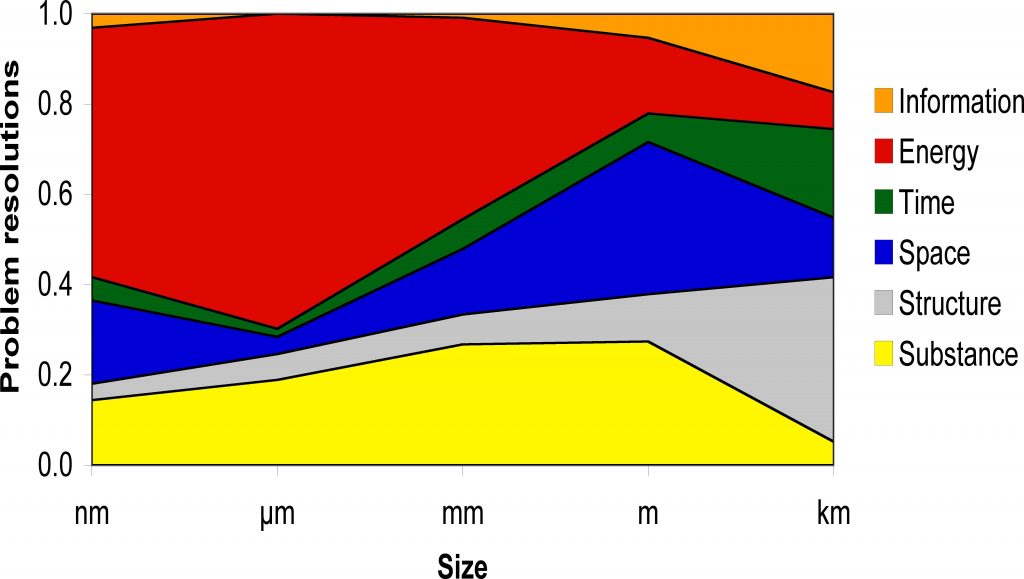
In technology (graph above), Energy rules, especially at the level of materials processing in which heterogeneity (related to Information) is destroyed to generate a homogeneous material with little specific Structure making it more easily manipulated during manufacture. Raw material (Substance) is largely taken from geological deposits.
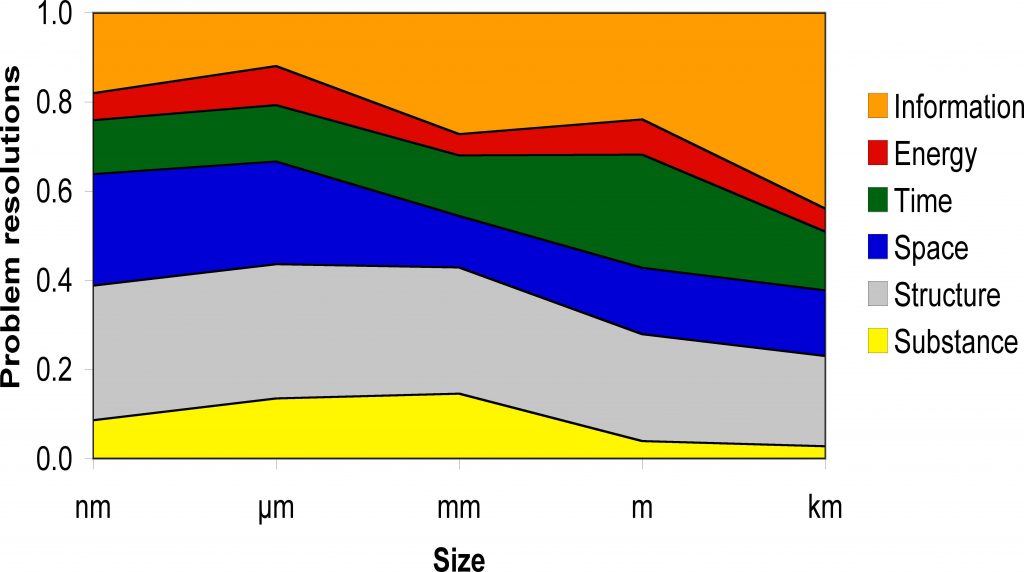
By contrast, Energy is hardly significant in biology (above), partly because all processing is done at ambient temperatures. Materials at the molecular level have sufficient Structure (derived from Information in the genetic system) to be able to ‘self-assemble’ (i.e. form complex structures, often – perhaps always – as liquid crystals), to deliver properties that rely on the combinations of polymers and minerals in specific structures. Raw material (Substance) is less significant for a number of reasons: ‘self-assembly’ introduces so many secondary bonds (H-bonds or weaker) that materials can be dismantled with less energy. Also the chemistry of life is much more uniform than that of technology, so the chemistry for recycling is far more general. Life would not be possible without significant recycling of the resources. We are all destined to die.
A significant feature of the biology graph is the way the six parameters form transverse bands, very different from technology. It seems likely that this is because multicellular organisms are just that – they are composed of populations of individual cells. These cells need to sense how they interact with the other cells in the organism and with the external environment, significantly requiring Information.
This article is based on one published in Professional Engineering, 2022, No 1.
Gordon, J E, and G Jeronimidis. 1980. “Composites with high work of fracture.” Philosophical Transactions of the Royal Society A 294:545-550.